Aurora Borealis – The Physics of our Polar Skies
You have probably heard of the breathtaking Northern Lights, or “aurora borealis.” They frequently occur in the very northern parts of Earth (around the South Pole, a similar phenomenon called the “aurora australis” occurs).
Often, the Borealis displays colors of violet, blue, green, yellow, red, and pink in the forms of arcs, rippling curtains, streamers, or eerie glows cast across the sky. While this aerial display is extremely beautiful and popular, the aurora borealis is more than pretty colors across the sky.
Like every other aspect of our universe, the natural phenomena is part of a complex system of relationships between atoms, light, and the atmosphere of the Earth that can be explained only by physics.
The Borealis starts at the surface of the sun when solar activity ejects a large cloud of plasma (ionized gas consisting of positive atoms and electrons) called a coronal mass ejection (CME). It takes approximately three days for one of these tiny gaseous particles to reach Earth’s magnetic field. The solar particles travel as an invisible, comet-shaped structure stretching a million miles from Earth in the direction opposing the Sun.
When the particles and Earth’s magnetic field collide, the magnetic tail of the comet undergoes complex chemical changes. These alterations generate currents of slightly negative or positively charged particles, which flow along Earth’s magnetic field lines into the Northern and Southern polar regions.
Boosted in energy, the gases collide with nitrogen and oxygen atoms from the upper atmosphere, producing a spectacularly breathtaking auroral light.
Distinctive patterns are created since these interactions occur only along Earth’s magnetic field lines. These patterns include pulses, steady glow, rippling curtains, or pulsating blobs, which together create the apparent texture of the Northern Lights.
The specific coloration of these eerily beautiful beams depends on both the type of atmospheric gas that the solar plasma collides with and the altitude of the interaction. For example, when plasma interacts with nitrogen, the colors red, violet, or blue may be produced while collisions with oxygen typically result in green or yellow.
However, altitude is the primary factor in coloration. Shades varying from powder blue to a deep, pulsing navy blue with hues of violet occur below 100 km (60 mi) above Earth’s surface, with bright lime to emerald green tints between 100-240 km (60-150 mi). Finally, tones of ruby red to vibrant scarlet are caused by the interaction of particles at an altitude above 240 km (150 mi).
The strength of the Northern Lights is measured in Kp, a numerical scale from zero to nine, also known as the planetary index. A Kp of zero is considered a very weak display, while nine is a powerful geomagnetic storm. Any area with a Kp of five or higher is considered a geomagnetic storm or a temporary disturbance of Earth’s magnetosphere.
To see the best display, it is recommended to visit locations with a high Kp value. Contrary to popular belief, the Borealis can actually be present during both night- and daytime, but is not visible to the naked eye when the sun has not set.
When going nocturnal aurora hunting, be sure to steer clear of locations with light pollution. Just like seeing the stars, it can become quite difficult looking for the auroras when in light-polluted areas. You can set out to Northern countries to catch a glimpse of the Borealis at any time of the year, on any day of the month.
However, while these stunning illuminations are a sight to see on anyone’s bucket list, they can also be disruptive to the electrical grids, power infrastructure, and satellites in space. Solar storms cause three waves of “punches” to electrical currents in man-made technology.
The first wave interferes with high-frequency radio communications and GPS signals, as the electromagnetic radiation first reaches Earth’s upper atmosphere, after about 8 minutes.
Scientists refer to the fast-moving particles that impact the space satellites as the second punch. When the gaseous particles hit Earth, they collide with the atmosphere so hard that portions of the plasma break off and can hit the satellites.
The CME creates the danger of the third punch. The billions of solar atmospheric material cause currents with high-voltage power lines when they interact with Earth’s magnetic field, which triggers protective relays in the power grid. In worst-case scenarios, such as the aftermath of the powerful solar storm in mid-March 1989, this leads to widespread blackouts.
Predicting these storms remains shrouded in mystery, and scientists are eager to further understand the physics of aurora and solar storms.
BONUS: To watch a video of the view of Earth from space click here. (Includes clouds, cities, thunderstorms, and the aurora borealis.)
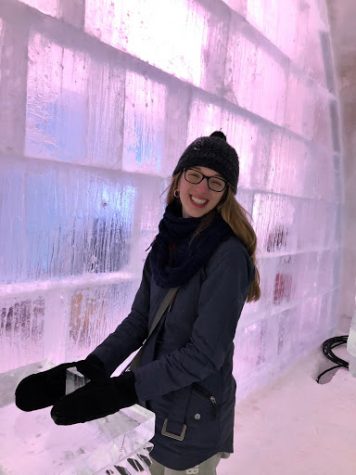
Kaelyn Klatte, a junior at Harriton, has been writing for The Harriton Banner since freshman year. An avid science fan, active Cross Country/track athlete,...