The Science Behind the Coronavirus PART ONE
An Interview with Biology and Chemistry Teacher Mr. MacNichol
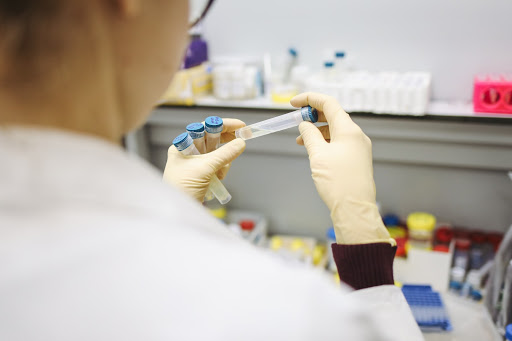
With the COVID-19 pandemic affecting hundreds of thousands of people every day, there’s no surprise that this disease is the subject of nearly every political discussion and has become the center of our attention.
Whether it be an infected loved one, the cancellation of an important upcoming event, or a switch to online learning, this virus has had an impact on almost everyone’s lives, young or old. Consequently, it is more important than ever for the public to understand the science behind this outbreak, so we can take action to prevent further escalation of the problem.
To better understand the science aspect of the COVID-19 virus, I interviewed (through email) Mr. MacNichol, a Harriton biology and chemistry teacher who is knowledgeable about the science of this disease. Let’s see what he has to say about this novel virus.
Why do the virus (SARS-CoV-2) and the disease (COVID-19) have different names?
SARS-CoV-2 stands for Severe Acute Respiratory Syndrome CoronaVirus 2, while COVID-19 stands for CoronaVirus Disease 2019. Since not everyone who is infected with the virus will necessarily present with the disease, scientists like to have a way to distinguish between these two scenarios. This is somewhat similar to the use of HIV vs. AIDS. Untreated infection with HIV, the human immunodeficiency virus , often leads to the development of the disease and secondary infections called AIDS, or acquired immunodeficiency syndrome.
What is a virus and how do viruses generally work?
All viruses have two primary components: (1) A nucleic acid genome that provides the instructions for making the viral proteins and (2) an outer shell that protects the virus and facilitates its entry into the cell. The nucleic acid genome can either be DNA (similar to our own) or RNA, like the mRNA molecules our cells use when making our own proteins. All viruses have a protein shell surrounding their genome called a capsid, which protects the genome while some viruses (like SARS-CoV-2) have an additional layer of lipids and proteins called an envelope that can be analogous to a cell’s membrane.
Regardless of the genome type and overall structure, all viruses eventually hijack your cell’s manufacturing system (ribosomes, ER, golgi, etc) for the purpose of their replication since they are nonliving and cannot replicate on their own. Once the virus commandeers your cellular machinery and replicates en masse, the virus exits the cell to infect other surrounding cells. This can be done through budding or lysis. Budding is the release of viral particles through exocytosis, similar to the way cells would secrete many cell products, while lysis is the complete rupture of the cell and the release of the viral particles.
How specifically does this virus, SARS-CoV-2, infect humans?
SARS-CoV-2 is a (+) sense RNA virus, meaning its genome is basically one large mRNA molecule. It has a protein nucleocapsid (N) that surrounds the RNA to protect it and then an additional envelope made up of a lipid layer with three significant embedded glycoproteins (E, M, and S) the most famous of which is the spike (S) protein. This spike protein is where coronavirus gets its corona name from (meaning crown), and it is also how the virus attaches to cellular proteins called ACE-2 receptors embedded in the membranes of the cells that line the branches of the lungs (bronchioles) and in the air-filled sacs at the ends of those branches called alveoli.
The spike protein uses a combination of polarity (yay chemistry!) and physical shape to adhere to the ACE-2 receptor — similar to the way a substrate fits into the active site of an enzyme. Unfortunately, for our current situation, the spike protein of the novel SARS-CoV-2 is actually a mutated form of the SARS-CoV-1 spike protein and has a greater affinity for our ACE-2 receptors. This means a smaller viral dose can lead to an infection taking root because viral infections are not a light switch, they are more like a dial so the concentration of virus you are exposed to matters.
By binding to the ACE-2 receptors, the virus then enters the cell in a vesicle by receptor-mediated endocytosis. Once inside the cell the virus will release its RNA genome into the cytoplasm. The infected cell reads this RNA as if it were mRNA producing the enzymes needed to make copies of the viral genome. Normally our cells have a good defense to stop all this viral production — an inhibitor protein called interferon — but SARS-CoV-2 keeps our cellular immune system at bay by inhibiting interferon production. The viral genome then transcribes individual mRNA strands for the production of each of the structural proteins that make up the virus [N, E, M, and S].
Making matters worse, the virus also quickly moves out of the cytoplasm (where it would be vulnerable) and into a double membrane network that connects the rough ER to the Golgi, free from our defense mechanisms. Here, the virus reprograms our already established manufacturing system — the rough ER and Golgi body — to stop building cellular proteins and focus primarily on the production of viral proteins and fully formed viruses. Once production is complete, the viruses are then shipped from the Golgi body in a vesicle much like any secretory protein would be shipped and released from the cell by way of exocytosis, moving on to infect other nearby cells.
Where did SARS-CoV-2 come from?
All life from humans to plants to bacteria can be infected by viruses. However any virus can usually only infect a few different species at most. We call pathogens that can be transferred between humans and animals (usually other mammals) zoonotic diseases. According to the CDC, 3 out of every 4 new emerging infectious diseases in people came from animals. Often these viruses exist in animals for a significant period of time before they develop some mutation that allows them to infect humans in what is called a spillover event. Since humans are mammals, we share many closely related cellular proteins with other mammals. This is why it often only takes a small, but significant, mutation to cause an animal virus to spill over into humans. This is why it’s so important for humans to not only limit the extent to which we disrupt wild populations but also closely monitor our livestock populations and practices.
Comparing the amino acid sequences of SARS-CoV-1 (2002), MERS (2012), and SARS-CoV-2 (2019), it is clear that bats are the primary source of all three of these coronaviruses. However, different intermediate animals likely transferred the virus from bats to humans. In the case of SARS-CoV-1 (2002), analysis suggests it was the civet [a cat-like mammal found in Africa and Asia], MERS (2012) a camel, and SARS-CoV-2 (2019) potentially a pangolin [scaly anteater]. This is not unique to SARS-CoV-2, in fact, the most common version of the seasonal flu endemic (H1N1) is a recent mutation of the 2009 swine flu. Zoonotic diseases and spillover events pose a great threat to humanity, because often the human population doesn’t have any acquired immunity, meaning the first few years of a novel virus can be devastating.
Why are bats the primary reservoir for coronaviruses, and do the viruses hurt the bats?
This is a very interesting question, and scientists have several hypotheses. First, [bats’] roosting behavior is the antithesis of social distancing. They often pack in very closely and for many different reasons. In the summer, female bats often maternity roost with their babies to protect the young; in the winter, bats form a hibernation roost to keep warm. This roosting causes them to easily spread diseases and viruses amongst themselves. However since bats are the only true flying mammals- the rest just fall with style – they have crazy high metabolic rates. So much so that they can have heart rates as high as 900 bpm and can use so much energy in flight that they risk daily starvation. This high metabolic activity may come with a hyperactive immune system, however, that prevents them from getting ill from the viruses they carry. [Due to] the lack of personal space, combined with the super immune system and the fact that some bats migrate 300+ miles in a season, they are uniquely primed to be the origin of some zoonotic spillover.
What are virus strains and how do new strains come about?
Ultimately viruses mutate a lot but the vast majority of those mutations make no noticeable difference from the original viral phenotype or actually make the virus less able to exist in humans, causing the mutation to peter out. Sometimes, however, a mutation will arise that changes some aspect of the virus’ phenotype and is stable enough to establish a new line of infection that avoids being selected against by natural selection. This is when scientists say the virus has mutated into a new strain.
The two primary ways new viral strains can come about is through antigenic drift and antigenic shift.
Antigenic drift is when small mutations to the viral genome come about through errors in their replication process and ultimately the antigen is functionally different enough to be classified as a new viral strain. Most viruses have a fairly regular mutation rate so scientists can estimate when these events will occur. SARS-CoV-2 has a substitution mutation rate of approximately two mutations per month. This is a relatively low mutation rate for an RNA based virus and so scientists feel comfortable that it will take several years for antigenic drift to cause a major change – which would be good news for the effectiveness of a vaccine once developed.
Antigenic shift is a large rapid change in the viral genome that comes from the virus merging genomes with another virus that infects your cell simultaneously. These events are harder to predict and can be very dangerous when they result in a stable virus that our immune system does not recognize.
SARS-CoV-2 is a respiratory virus — what happens to the respiratory system in an individual with COVID-19?
SARS-CoV-2 can actually affect many cells in your body that possess the ACE-2 receptor. This is why SARS-CoV-2 is somewhat unique in being a virus that infects both your upper respiratory tract as well as the lower respiratory tract. Typically upper respiratory infections are more contagious but less serious, while lower respiratory infections are more serious but less contagious. Some researchers hypothesize that this infection starts mildly in the upper respiratory tract and is very contagious while most people don’t realize they are infected, and it is not until it processes down to the lower tract that the more serious symptoms begin to show yet the transmission damage has already been done.
To understand how the serious symptoms arise you first need some background. The main structure in the lower respiratory tract are these tiny air filled sacs called alveoli – the primary tissue responsible for gas exchange between your lungs and blood capillaries. Alveoli have two major cell types (pneumocytes 1 and 2) with type 1 responsible for gas exchange and type 2 responsible for the production of a really important chemical called surfactant. Surfactant is an amphipathic (both polar and nonpolar) mixture of lipids and proteins that lines the inside of your alveoli. This liquid is responsible for preventing the water that lines your alveoli from sticking together. Without surfactant the sides of the alveoli would attract each other, stick together, and essentially make it so that you could not expand your lungs and breath. You may already see where this is going.
When SARS-CoV-2 infects the type 2 pneumocytes it hijacks their manufacturing pathway and forces it to produce viral particles instead of surfactant. Now under attack, the cells start to alert the immune system of the infection by presenting the spike antigen on the surface of their cells and releasing immune messengers called cytokines. These cytokines trigger local inflammation, tell the hypothalamus to turn up the dial on your temperature, and get the ball rolling on your more advanced immune processes. Normally this response would be just what the doctor ordered. However, an inflammatory response brings extra fluid, which when paired with the lower production of surfactant, leads to your alveoli to be stickier than normal. This initially presents itself as a shortness of breath and a dry cough since your airway isn’t actually blocked by mucus but rather your alveoli are just sticking together and not filling back up with air. At this point the patient notices the characteristic dry cough and fever that suggest you’ve been infected with SARS-CoV-2.
I feel it is important to pause and point out that the vast majority of people experiencing these symptoms will develop an appropriate immune response and fully recover in a few weeks.
In some patients however, the inflammation in the lungs can progress to the point where the patient will develop pneumonia, have difficulty breathing, and need to be hospitalized. Still, at this point, many patients recover.
For a few (~2%) the immune system dramatically escalates the situation by flooding the lungs with immune cells to clear away the damage and repair the lung tissue. When working properly, this inflammatory process is tightly regulated and confined only to infected areas. But sometimes your immune system goes haywire, enlisting the help of cytotoxic T-cells which kill anything in their way, including your healthy tissue. This comes with an increased release of cytokines, as well as increased inflammation and permeability of the lung tissue. This all leads to severe lung damage and ARDS, and by this point a person cannot breathe on their own without the use of a ventilator. Very little oxygen is being absorbed into the bloodstream, and because of all the inflamation, the patient’s blood pressure is very low. This makes it hard to get oxygen to many vital organs, most notably the liver with acute liver disease and in some cases septic shock. Many people that progress to this point unfortunately do not make it.
How does an infection cause a person to develop immunity?
Generally when a virus infects your cell, the cell will take viral proteins (called antigens) and place them on the surface of the cell to alert the immune system of the intruder. In the case of SARS-CoV-2, this antigen is the famous spike protein. When a cell presents this antigen on its surface, it initiates a complex immune response that leads to immune cells, called B cells, multiplying rapidly. These B cells become factories for the production of a powerful molecular weapon called an antibody. Antibodies are able to chemically bind with the antigen and effectively neutralize them. In the case of SARS-CoV-2’s spike antigen, antibodies would neutralize the ACE-2 receptor binding site on the spike protein, preventing the virus from infecting new cells. With the viruses now trapped outside of the host cell, general macrophage white blood cells come along and gobble them up.
As effective as this process can be, it unfortunately takes a while to initiate for the first time. The good news is once your body makes specific B cells for an infection it tends to keep a bunch of them around in case the virus returns. The response to a secondary infection is usually so fast that you never even knew it occured. This is what we call immunological memory and it is the basis of immunity.
Can you get infected with the SARS-CoV-2 twice?
This is a really important question and unfortunately the answer is that it’s too early to be certain. Often when you are infected with a virus you gain some level of immunological memory, which limits a reinfection. However, that memory can last anywhere from months to a lifetime. Since this virus is so new, it hasn’t been around long enough to really have a clear picture. Some promising research from a team of Chinese researchers that exposed rhesus monkeys to the virus showed several weeks after the monkeys had recovered from the virus they still had high levels of the protective antibodies in their system, suggesting a good short term immunological memory. However, a 6 year follow-up study of individuals infected with the first SARS virus showed that none of the 23 individuals with positive infections had any detectable SARS specific memory B cells remaining. This fits with other studies from that time that suggest the immunity to SARS-1 likely lasted only about 1 year. It’s important to mention that this is not SARS-1 even though SARS-1 and SARS-2 both come from the same viral evolutionary branch. Only time will tell.
Watch for PART TWO of this exclusive Banner series.
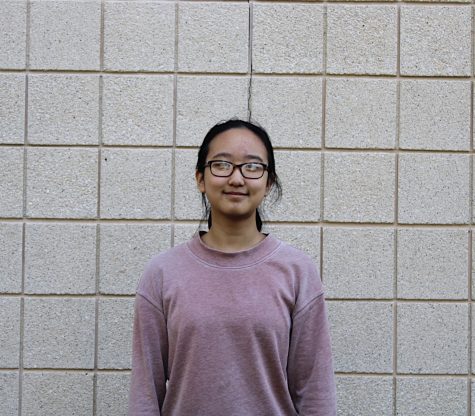
Hannah, a senior, is excited for her fourth year in the Harriton Banner! As a returning Science & Tech section editor, she is excited to continue reading...